Parasites & Vectors volume 15, Article number: 422 (2022 ) Cite this article
Mosquitoes are responsible for disease transmission worldwide. They possess the ability to discriminate between different ecological resources, including nectar sources, animal hosts and oviposition sites, a feature mediated by their olfactory system. Insect repellents, such as N,N-diethyl-meta-toluamide (also called DEET), have been shown to activate and inhibit mosquito odorant receptors, resulting in behavioral modulation. This and other repellents currently available for personal protection against mosquitoes are topically applied to the skin and operate at a short range. In our search for potential long-range inhibitors of attractants to human hosts, we have hypothesized that the shared chemical similarities between indole and DEET may confer the former with the ability to block odorant receptor function and inhibit human host attraction in a similar way as DEET. Zinc Pyrithione For Seborrheic Dermatitis
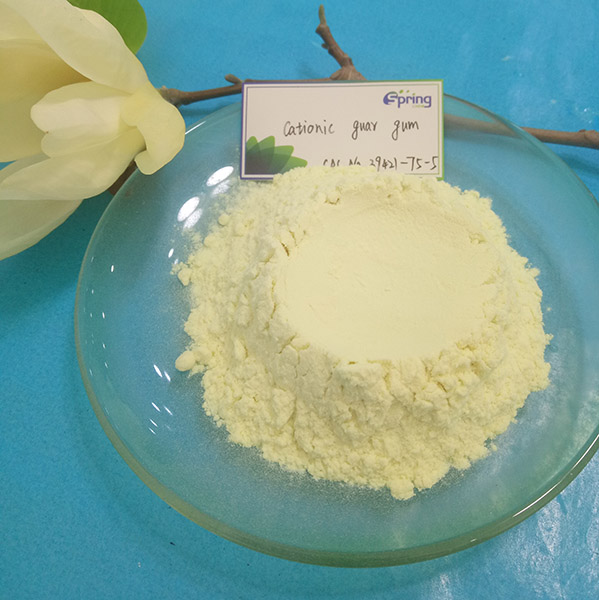
We used the two-electrode voltage clamp system to assay Xenopus laevis oocytes as a platform to compare the pharmacological effect of commercially available insect repellents and indole on the Aedes aegypti (R)-1-octen-3-ol receptor, OR8, a receptor involved in the decision-making of female mosquitoes to identify human hosts. We also conducted arm-in-a-cage and wind-tunnel bioassays to explore the effect of indole on human host-seeking female Aedes aegypti mosquitoes.
Our results demonstrate that indole inhibited the Aedes aegypti (R)-1-octen-3-ol receptor OR8. In our arm-in-a-cage assay, 1 M of DEET reduced mosquito visits on average by 69.3% while the same indole concentration achieved 97.8% inhibition. This effect of indole on flight visits was dose-dependent and disappeared at 1 μM. In the flight tunnel, indole elicited on average 27.5% lower speed, 42.3% lower upwind velocity and 30.4% higher tortuosity compared to the control.
Indole significantly inhibits OR8 activation by (R)-1-octen-3-ol, mosquito visits to a human hand and long-range human host-seeking. The volatility of indole may be leveraged to develop a novel insect repellent in the context of personal mosquito protection.
Mosquitoes are a major vector of infectious diseases worldwide due to their ability to transmit pathogens and are also a major source of annoyance. A spatial repellent, such as N,N-diethyl-meta-toluamide (DEET), inhibits mosquito attraction at various distances from the host, ranging from a few centimeters to close to 1 m [1,2,3]. However, despite the protection such repellents provide, mosquitoes are still able to efficiently locate potential human hosts, hover around them and locate any unprotected area that can be targeted for biting. It is therefore desirable to find alternative solutions with an extended protection range, such as volatile odorant repellents [1]. Plant volatiles, such as geraniol, citral, citronellal, eugenol and anisaldehyde, have been shown to exhibit spatial repellency against mosquitoes [4]. In the future, these plant odorants may play a major role in the development of spatial repellent technology.
Mosquito repellents such as DEET exhibit low volatility and have been reported to act at ranges of between 4 and 8 cm when used in cages [2]. These compounds exhibit complex interactions with odorant receptors (ORs), activating some ORs while inhibiting others [5,6,7]. Notwithstanding its odor masking properties [8,9,10], DEET alone activates the Aedes aegypti indole-sensitive receptor AaegOR2 and also inhibits the (R)-1-octen-3-ol-activated receptor AaegOR8 [5]. The OR8 gene is expressed in basiconic sensilla on the maxillary palps of mosquitoes belonging to species of the Anopheline and Culicine families [11, 12]. This phylogenetically conserved receptor specifically responds to the (R)-1-octen-3-ol enantiomer in the nanomolar range [13, 14] and is believed to synergize the effect of CO2 in the attraction to human hosts [15,16,17].
Indole alone does not mediate oviposition in Anopheles gambiae (Diptera: Culicidae) [18] and Ae. aegypti [19] unless combined with 3-methyl-1-butanol in An. gambiae [18] and nonanal, p-cresol, phenol and dimethyltrisulfide in Culex spp. (Diptera: Culicidae) [20]. High indole dosages have been demonstrated to repel the oviposition of mosquitoes such as Toxorhynchites spp. (Diptera: Culicidae) [21], Culex quinquefasciatus [22], Aedes spp. [19] and An. gambiae [23].
Noting that DEET and indole exhibit overlapping chemical features, including the presence of an aromatic ring and a neighboring nitrogen atom (Fig. 1a), we hypothesized that indole might interfere with OR8 function. In the present study we used the two-electrode voltage clamp method to assay Xenopus laevis oocytes expressing Ae. aegypti OR8 and showed that indole inhibited OR8-mediated current depolarizations elicited by (R)-1-octen-3-ol. We also showed that sustained exposures to indole and repellents generated an increase of the current baseline. We observed that co-administration of indole and (R)-1-octen-3-ol evoked significant hyperpolarizations that were concentration dependent. In light of these pharmacological results, we tested the potential behavioral effect of indole on animal host-seeking female Aedes aegypti using an arm-in-a-cage assay, providing evidence that indole acted as a repellent at various doses. Using a wind tunnel, we showed that indole decreased anemotactic flight elicited by a synthetic human host blend composed of CO2 and 1-octen-3-ol. Our findings suggest that volatile compounds such as indole may be discovered based on pharmacological knowledge of ORs. The volatility of indole and the phylogenetic conservation of the CO2 and octenol receptors in the Culicidae family may be leveraged to develop a novel insect repellent with a broad spectrum of action in the context of personal mosquito protection.
The mosquito laboratory-reared colony developed by Prof. Joel Margalit was maintained in an air-controlled insect chamber at 26 °C, 80% relative humidity (RH) and 12:12-h light/dark photoperiod. Mosquito larvae were kept in plastic pans containing 1 l of water. Larvae were fed with a ground mixture of Novocrabs feed (JBL GmbH & Co., Neuhofen, Germany), mice feed and dry yeast until adult emergence. Pupae were transferred into plastic cages. and eclosed adults were allowed to mate and feed ad libitum on a 10% sucrose solution. Females were fed with cow blood using a membrane feeding system.
In vitro transcription and two-microelectrode voltage-clamp electrophysiological recordings were carried out as previously described [14]. Both the AaegOR8 OR and the Ae. aegypti odorant coreceptor (AaegOrco) were synthesized using the mMESSAGE mMACHINE® SP6 kit (Thermo Fisher Scientific, Waltham, MA, USA) from the linearized pSP64tRFA expression vector. Xenopus laevis oocytes were manually collected, separated and treated for 30 min with a 8 mg/ml collagenase solution at 18 °C to remove the follicular layer. Stage V–VI oocytes were rinsed in Ringer solution (96 mM NaCl, 2 mM KCl, 5 mM MgCl2 5 mM HEPES, pH 7.6) and microinjected with a mixture of 1 μl AaegOR2 (3 μg/μl), 1 μl AaegOrco (3 μg/μl) and 1 μl of double-distilled water. Injected oocytes were maintained at 18 °C for 3 days in Ringer’s solution (96 mM NaCl, 2 mM KCl, 5 mM MgCl2, 0.8 mM CaCl2, 5 mM HEPES, pH 7.6) supplemented with 5% dialyzed horse serum, 50 μg/ml tetracycline, 100 μg/ml streptomycin and 550 μg/ml sodium pyruvate.
Whole-cell currents were monitored and recorded using the two-microelectrode voltage-clamp technique. Holding potential was maintained at − 80 mV using an OC-725C oocyte clamp (Warner Instruments, LLC, Hamden, CT, USA). Oocytes were placed in a RC-3Z oocyte recording chamber (Warner Instruments, LLC) and exposed for 8 s to different concentrations of indole (CAS 120-72-9; Sigma-Aldrich, St. Louis, Mo, USA), (R)-1-octen-3-ol (CAS 3687-48-7, 98.2%; Bedoukian Research Inc., Danbury, CT, USA), N,N-diethyl-m-toluamide (DEET; CAS number 134-62-3; Sigma-Aldrich) and the insect repellent ethyl butylacetylaminopropionate (IR3535; CAS 52304-36-6; Merck & Co., Inc., Kenilworth, NJ, USA). All compounds were solubilized in 200 μl of dimethyl sulfoxide (DMSO) prior to dilutions in Ringer’s buffer. Orco alone was expressed in oocytes and exposed to N-(4-ethylphenyl)-2-{[4-ethyl-5-(pyridin-3-yl)-4H-1,2,4-triazol-3-yl]sulfanyl}acetamide (VUAA1; Innovapharm Ltd., Royal Sutton Coldfield, UK) as a positive control. Currents were allowed to return to baseline between odorant applications. Data acquisition were carried out with a Digidata 1550A and pCLAMP10 (Molecular Devices, Sunnyvale, CA, USA). Plotting and statistical analyses of the data were performed using GraphPad Prism 7 (GraphPad Software Inc., La Jolla, CA, USA). To determine the sensitivity of OR8 towards the different indole treatments, we interpolated the half-maximal effective concentration (EC50) values, which correspond to the ligand concentrations eliciting 50% of the maximal current response.
To investigate the potential role of indole in human host-seeking behavior, we developed an arm-in-cage bioassay in which the experimenter’s hand is presented to fifteen 5–10-day-old post emergence adult females. The bioassay comprises custom-designed three-dimensional-printed interlocking ring that creates a 55-mm-diameter opening in a powder-free latex glove worn by the experimenter. A plastic screen is wedged between the two ring components to protect against mosquito bites. The ring supports an odorant delivery platform located at the center of the ring, which receives a 10-mm-diameter cover glass and two 5-mm-diameter filter discs (WHA10016508; Merck) (Additional file 1: Figure S1, Additional file 2, Additional file 3). Mosquitoes are introduced in a 20.3-cm3 metal cage located inside an incubator for at least 10 min before each experiment (26 °C, 80% RH) for acclimatation. This cage was chosen based on an earlier study reporting the effects of repellents on flight approach in Ae. aegypti [2]. The cage is then placed at room temperature (23–25 °C) under a video camera (EOS 70D, lance: MACRO 0.25/0.8ft; Canon Inc., Tokyo, Japan) to monitor and quantify the numbers of mosquito visits and their duration on the plastic screen. We used the solvent diethyl ether (DEE) as a vehicle. On a blank filter disc, 25 μl of DEE, indole or DEET was deposited on the filter paper and allowed to evaporate for 2 min prior to mosquito exposure. We used tenfold dilutions of indole ranging from 10–6 to 10–1 M, and 10–1 M DEET. The experimenter rubbed the ring-mounted glove against the shirt and skin. All experiments were conducted during the first 3 h of the diurnal period and lasted 10 min. This schedule was chosen for practical reasons and also because mosquitoes consistently exhibited attraction to the human hand. To determine the repellency of indole against human host-seeking mosquitoes, we recorded the number of females landing per minute and counted the duration of their stay on the screen surface. Mosquito landing count was normalized (\({x}^{^{\prime}}= \frac{x-\mathrm{min}(x)}{\mathrm{max}\left(x\right)-\mathrm{min}(x)}*100)\) . Mosquitoes returning to the region of interest were counted as a new visit. Statistical comparisons of mosquito landing were carried out with a Kruskal–Wallis H-test and post-hoc pairwise comparisons were carried out using the Wilcoxon rank sum exact test (P-value adjustment method: Benjamini-Hochberg [BH]). A one-way analysis of variance (ANOVA) analysis was used to analyze mosquito duration.
A wind tunnel system was used to measure the response of female Ae. aegypti to CO2 alone, 1-octen-3-ol and indole stimuli. The wind tunnel is a rectangularly shaped chamber (2200 × 500 × 500 mm) composed of three compartments: a release, flight and odor delivery chamber, respectively. The release chamber holds the mosquitoes prior to the experiment, while the odorant chamber releases an odor from a clean air delivery system (Sigma Scientific LLC., Micanopy, FL, USA). Ten females (same age as previously described) were transferred to a release box (200 × 160 × 200 mm) inside the release chamber (500 × 200 × 500 mm) and allowed to acclimate for 1 h in darkness at 27 ± 0.7 °C. Experiments were conducted between 10:00 and 13:00 local time. Three experiments were conducted for each treatment. The flight tunnel (1500 × 500 × 500 mm) was illuminated with five infra-red lights (RT VAR2-i2-1; Raytec Ltd., Ashington, UK), and a laminar air flow was applied at a speed of 0.1 m/s. After acclimatization, the injection air flow was manually opened for 15 min (duration of each experiment) with compressed air (4 l/m), which delivered the stimulus. Immediately afterwards, flight coordinates were recorded using TrackIt system (SciTrackS GmbH, Pfaffhausen, Switzerland) and the release box door opened. The time interval between each experiment was at least 30 min under constant room ventilation with the wind tunnel top cover removed. Manual maintenance of the wind tunnel was carried out using nitril latex-free gloves to prevent odor contamination.
Flight trajectories were recorded using two acA2000-165um infrared-sensitive cameras (Basler AG, Germany) and processed by the 3D software TrackIt (SciTrackS GmbH, Switzerland). Output data was analyzed through R version 4.0.2 programing language [24]. A description of the functions to quantify parameters of flight trajectories can be found in Additional file 4: Table S1. In total, the combined treatments consisted of 90m614 raw X, Y, Z coordinates. Coordinates located outside the flight tunnel (reflection) were removed. The visual field was cropped to define a 800 × 500 × 500 region of interest (ROI; Fig. 5a). Coordinates within the first 3 min of recording were retained and analyzed. We randomly sampled 25% of the remaining coordinates (5192 coordinates) for statistical analysis. Statistical comparisons between treatments were carried out with a Kruskal–Wallis H-test, and post-hoc pairwise comparisons were carried out using the Wilcoxon rank sum exact test (P value adjustment method: Bonferroni).
The injected stimuli included: (i) CO2 (600 ± 20 ppm) + 25 µl of DEE; (ii) CO2 + 25 µl of DEE + 2 µl of 1-octen-3-ol (168 mg); and (iii) CO2 + 25 µl of DEE + 2 µl of 1-octen-3-ol (168 mg) with indole 5 M (14.625 mg). Each stimulus was deposited on a double Whatman filter paper (WHA10016508; Merck & Co., Inc.) as described above in the arm-in-cage bioassay. To allow DEE to evaporate, the holder was placed for 2 min in a separate room, following which the chemical holder was placed inside an OSI-4550 inline volatile collection chamber (Sigma Scientific LLC) connected between the clean air delivery system and the injector. CO2 was delivered by a steel compressed gas cylinder, and its content was monitored at the injector site using a TES-1370 CO2 analyser (TES Electrical Electronic Corp., Taipei, Taiwan). During all these procedures, the person conducting the experiment wore laboratory gloves and a mask.
Based on the chemical similarities between indole and DEET (Fig. 1a), we surmised that indole would exert an inhibitory effect on AaegOR8. A 10–3 M indole concentration reduced by approximately 30% the AaegOR8 current amplitude elicited by 10–7 M (R)-1-octen-3-ol (Fig. 1b). This effect was not observed in Orco-injected oocytes.
Indole inhibits OR8-Orco activation by (R)-1-octen-3-ol. a (R)-1-octen-3-ol is an alkenyl alcohol and a potent ligand of OR8. Indole is an aromatic bicyclic organic compound. DEET is a benzyl-ester sharing a benzene ring and a nitrogen atom with indole (red features). b Representative current traces of AaegOR8-Orco activation by 10–7 M (R)-1-octen-3-ol (black arrowheads) or by a mixture of 10–3 M indole (red arrowhead) and 10–7 M (R)-1-octen-3-ol (black arrowhead). Normalized responses of AaegOR8-Orco to 10−7 M (R)-1-octen-3-ol alone (control) or in combination with 10−3 M indole (indole). Statistical significance was determined using the Wilcoxon–Mann–Whitney test (n = 5–6). Orco-injected oocytes responded to 10–3 M of the Orco agonist VUAA1 (positive control) but not to 10–3 M indole (n = 4). c Representative current traces of AaegOR8-Orco activation by (R)-1-octen-3-ol alone or in the presence of 10–4 M, 10–3 M or 10–2 M indole. Arrowheads above the traces indicate the onset of the odorant stimulus, black arrowheads represent (R)-1-octen-3-ol and red arrowheads represent indole. d Concentration–response relationships of AaegOR8-Orco in response to increasing indole concentrations. Current amplitudes were normalized to the maximum response. Statistical significance was determined using a one-way analysis of variance (ANOVA; P = 0.0142) followed by a Dunn’s multiple comparisons test (n = 4–7). e Non-normalized concentration-current response relationships (n = 4–7). f Histogram of the depolarization current response amplitudes of AaegOR8-Orco in response to (R)-1-octen-3-ol alone (black) or in combination with various indole concentrations (shades of red). Statistical significance was determined using the multiple t-test and by the Holm-Sidak method (*P ≤ 0.05; **P ≤ 0.01; ***P ≤ 0.001; n = 3–7). g Concentration–response relationships of indole-induced hyperpolarization currents exhibited by AaegOR8-Orco-injected oocytes. AaegOR8-Orco, Aedes aegypti odorant receptor 8-odorant coreceptor; DEET, N,N-Diethyl-meta-toluamide
To determine the nature of this inhibitory effect, we established a series of concentration–response curves using OR8-Orco-injected oocytes exposed to increasing concentrations of (R)-1-octen-3-ol alone or combined with 10–4 M, 10–3 M or 10–2 M indole (Fig. 1c). Two types of currents were observed, including the expected agonist-induced depolarization currents and unusual indole-dependent hyperpolarization or reduction in baseline currents.
The interpolated EC50 values for octenol alone versus 10–4 M and 10–3 M indole were not statistically significant (Fig. 1d; Additional file 5: Table S2). The EC50 value elicited by 10–2 M indole was significantly different from that elicited by (R)-1-octen-3-ol alone, but the response was moderate. These findings suggest that indole does not have an important effect on the sensitivity of this receptor for (R)-1-octen-3-ol. Contrary to our initial results (Fig. 1b), we did not observe any significant inhibition of the amplitude response with increasing indole concentration (Fig. 1e). However, current amplitudes are contingent on oocyte inherent variability. To address this limitation, we determined the systematic effect of indole on depolarization current amplitudes by normalizing all of the current responses to the initial 10–7 M (R)-1-octen-3-ol exposure (Fig. 1c). We noted that 10–2 M indole consistently reduced the current amplitude across the concentration of (R)-1-octen-3-ol, excluding 10–10 M and confirmed that 10–3 M indole significantly reduced the response amplitude of AaegOR8 to 10–7 M (R)-1-octen-3-ol (Fig. 1f).
Indole concentrations of 10–3 M and 10–2 M elicited hyperpolarization currents (reductions in current baseline) in the presence of (R)-1-octen-3-ol concentrations ranging from 10–10 M to 10–7 M (Fig. 1c). These hyperpolarization currents were concentration dependent and were surmounted at higher (R)-1-octen-3-ol concentrations (Fig. 1g). To understand the contribution of indole alone to these currents, we exposed OR8 to increasing concentrations of indole without any other ligand present (Fig. 2a) and observed that 10–6 to 10–4 M indole evoked small depolarization currents. The two highest indole concentrations elicited either depolarization or hyperpolarization currents that were a fraction of those elicited by the initial 10–7 M (R)-1-octen-3-ol stimulation. To better characterize these small yet inconsistent effects, we focused on the currents elicited by 10–2 M indole and consistently observed these small hyperpolarization and hyperpolarization currents (Fig. 2b). By comparison, this same concentration of indole, in the presence of (R)-1-octen-3-ol at concentrations as low as 10–10 M, elicited currents reaching the initial response to 10–7 M (R)-1-octen-3-ol, suggesting that the significant indole-induced hyperpolarization currents require the presence of (R)-1-octen-3-ol (Fig. 2c).
High indole concentrations elicit both depolarization and hyperpolarization currents. a Representative current traces of AaegOR8-Orco activation by 8-s-long stimulations of (R)-1-octen-3-ol alone or increasing indole concentrations (10–6–10–2 M). Arrowheads above the traces indicate the onset of the odorant stimulus, black arrowheads represent (R)-1-octen-3-ol and red arrowheads represent indole. b Representative current trace of AaegOR8-Orco activation by 10–7 M (R)-1-octen-3-ol (black arrowhead) and by 10–2 M indole (red arrowhead). Statistical significance was determined using the Wilcoxon–Mann–Whitney test (n = 7). c (R)-1-Octen-3-ol was required to elicit significant indole-induced hyperpolarization currents. Nanomolar concentrations of (R)-1-octen-3-ol induced larger indole-induced depolarization currents than indole alone. Representative traces are shown above. Statistical significance was determined using a t-test (n = 7–12)
The cause of the observed hyperpolarization currents caused by indole in the presence of (R)-1-octen-3-ol required further investigation. It mirrored a phenomenon previously documented with AaegOR8, AaegOR2 and AaegOR10 [5, 7] and more recently with additional ORs from Cx. quinquefasciatus, Ae. aegypti, and An. gambiae [25]. To investigate whether the hyperpolarization current was a transient response or a durable modification of the current baseline, we exposed OR8-injected oocytes to a change of perfusion buffer by switching from the ND96 buffer solution to a 5.10–3 M indole perfusion. We also administered increasing 10-fold dilutions of (R)-1-octen-3-ol before reversing the perfusion solution back to ND96 (Fig. 3a). Prior to and after the exchange of the two perfusion buffers, the oocyte was exposed to a transient stimulation of 10–7 M (R)-1-octen-3-ol for control purposes. The switch from ND96 buffer to indole elicited a stable decrease in the baseline current not observed in water-injected oocytes (Fig. 3a). (R)-1-Octen-3-ol produced very little depolarization current at all tested concentrations. By comparison, DEET and IR3535 evoked larger currents. The switch in buffers in the opposite direction exhibited a stable decrease in baseline current that was most pronounced in the case of DEET and IR3535 (Fig. 3a). In these experiments, we treated the oocytes with a lower indole concentration, as compared to DEET and IR3535, because 10–2 M indole consistently killed the perfused oocytes. All observed currents elicited by (R)-1-octen-3-ol, indole, a mixture of these two ligands and buffer switch are summarized in Fig. 3b.
Indole and insect repellents (DEET, IR3535) increased the baseline current state of AaegOR8-Orco-injected oocytes. a Representative current traces of AaegOR8-Orco successively exposed to 100 nM (R)-1-octen-3-ol in ND96 buffer (saline; black trace) and continuous perfusion under indole (0.005 M), DEET (0.01 M) and IR3535 (0.01 M) perfusions (red traces), with increasing concentrations of (R)-1-octen-3-ol, ND96 buffer perfusion and 100 nM (R)-1-octen-3-ol. The same protocol was also applied to water-injected oocytes. In AaegOR8-Orco-injected oocytes, perfusion buffer transitions from ND96 buffer to indole, DEET or IR3535 are shown as solid red arrows, while the transitions in the reverse direction are shown as a solid black arrow. In water-injected oocytes, the corresponding buffer transitions are indicated by empty red and black arrows. The baseline currents of OR8-Orco-injected oocytes exhibited significant increases and decreases compared to those of water-injected oocytes. Statistical significance was determined using the Wilcoxon–Mann–Whitney test (n = 5–9). b Under saline perfusion (ND96), AaegOR8-Orco elicitd a stable current baseline. A stimulus pulse of (R)-1-octen-3-ol activated AaegOR8-Orco, which translated into a transient whole-cell depolarization current. A pulse of (R)-1-octen-3-ol (low concentration) and indole (high concentration) mixture transiently induced a hyperpolarization current. Increasing (R)-1-octen-3-ol concentrations reversed indole blockade. High indole concentrations elicited small depolarization and hyperpolarization currents. Under indole perfusion, the current baseline was increased. IR3535, Ethyl butylacetylaminopropionate
To explore the behavioral role of indole, we exposed a human hand to female mosquitoes using an arm-in-a-cage assay (Fig. 4a insert). The hand was covered with a protective glove which allowed mosquitoes to detect human skin odor through a window created by an open area on the dorsal side of the hand (Additional file 6: Video S1). This open area was protected by a screen and was equipped with an odor delivery system (Additional file 1: Figure S1, Additional file 2, Additional file 3). Increasing doses of indole ranging from 10–6 to 10–1 M were deposited on this delivery system, and repellency was measured in terms of number of mosquito visits and duration of visits. The repellency effect of DEET was significantly higher than that of the DEE vehicle and indole 10–6 M, respectively (Fig. 4a) (Kruskal–Wallis H-test, H = 106.11, df = 8, P < 0.0001). All treatments with indole, except for 10–6 M, were significantly different those with the vehicle (Fig. 4a). Increasing indole doses reduced the number of mosquito visits from 40.6% to 93.8%. We observed a 3.6% inhibition with a 10–6 M indole, but this effect was not statistically significant. Looking at the accumulated landing numbers, DEE vehicle and 10–6 M indole elicited overlapping temporal dynamics (Fig. 4b). Indole at 10–1 M had a significantly higher temporal repellency than all other treatments, including DEET at the same concentration. Other indole treatments exhibited intermediate temporal repellency between these two extremes. However, the only significant differences were observed between the vehicle and 1 M indole (Additional file 7: Fig. S2). In terms of visit durations, mosquitoes spent on average the same amount of time on the open area when landing occurred (Fig. 4c) (ANOVA, F(8,18) = 1.219, P = 0.343).
Indole repels human-seeking female Aedes aegypti mosquitoes. a Diagram of the arm-in-cage assay (inset). The gloved had of the person participating in the experiment is introduced inside a cage containing 15 Ae. aegypti female mosquitoes. The exposed skin area was monitored by a recording camera (see Additional file 6: Video S1 for a representative video). The number of mosquito visits were counted and normalized as a function of treatments, including treatments with the vehicle (diethyl ether [DEE]), DEET 1 M and 7 indole concentrations. All treatment groups were analyzed using a Wilcoxon rank-sum test (n = 3, see Methods section for more details). The percentage of inhibition is indicated below the graph in the gray shaded area. Different lowercase letters above bars indicate significant difference at P ≤ 0.05). b Hyperbola line fitting of the cumulative number of mosquito landings over time. Points represented are mean ± standard deviation of the mean (n = 3). See Additional file 7: Fig. S2. c Histogram of the normalized average time duration mosquitoes spent on the exposed skin area. A one-way ANOVA analysis was used to analyze all treatment groups. The Shapiro–Wilk normality test (W = 0.95056, P = 0.2212) was performed on the square root of the dependent variable (n = 3). Vertical lines indicate SEM
The results of the present study up to this point provided circumstantial pharmacological evidence that indole may in part affect OR8-mediated detection, but they did not give any direct indication that indole affects 1-octen-3-ol-mediated attraction in the context of human host-seeking. To explore this possibility, we used a flight tunnel (Fig. 5a) to expose human-host seeking female mosquitoes to a synthetic blend composed of CO2 and 1-octen-3-ol. We used three odor treatments, including CO2 alone or in combination with 1-octen-3-ol and indole, respectively. The ROI was divided into three sections (ROI-1, 2, 3; Fig. 5a) to explore possible differences in terms of trajectory speed, velocity and tortuosity. Kernel density estimations of mosquito locations along the X-axis were statistically different between the three treatments (Fig. 5b). A bird’s-eye view (X–Y plane) of flight trajectories representing flight speed suggested differences between the treatments (Fig. 5c; see example of individual trajectories in Additional file 8: Fig. S3). 1-Octen-3-ol seemed to increase the number of trajectories and coverage of the ROI while indole appeared to reduce flight speed across that same area. Among all three sections of the ROI, speed was higher and more consistent in response to CO2 in ROI-1 and -2 than in ROI-3 (Fig. 5d) (Kruskal–Wallis H-test, H = 86.8, df = 2, P < 0.0001). As a result, we focused on ROI-1 and -2 for further analyses. As reflected in Fig. 5c, higher speeds were elicited with the addition of 1-octen-3-ol than with CO2 alone (Fig. 5e). The addition of indole elicited significant decreases in speed compared to those observed with CO2 alone or those in combination with 1-octen-3-ol (Kruskal–Wallis H-test, H = 301.2, df = 2, P < 0.0001). While upwind velocity did not show any statistical differences between CO2 and 1-octen-3-ol, indole elicited lower upwind velocities (Fig. 5f) (Kruskal–Wallis H-test, H = 35.5, df = 2, P < 0.0001). Finally, indole-induced tortuosity was significantly higher than the tortuosity elicited by CO2 alone or in combination with 1-octen-3-ol (Fig. 5g) (Kruskal–Wallis H-test, H = 20.9, df = 2, P < 0.0001).
Indole reduces anemotactic behavior induced by CO2 and 1-octen-3-ol. a Schematic of the flight tunnel. The ROI was equally divided into three sections represented by differentially shaded volumes (ROI-1, -2, -3). b Kernel density estimations of mosquito coordinates within the first 3 min of odorant-induced flight in ROI-1, -2 and -3. Statistical significance is given in parentheses (P ≤ 0.05). c Bird’s-eye view of the collective flight trajectories elicited by the three odor treatments. Each visible dot represents a trajectory coordinate. Speed levels along the trajectory are color-coded according to the speed index (mm/s). Graded areas below the X-axes represent the three sections of the ROI (1, 2, 3). d Violin plot of mosquito speed in ROI-1, -2 and -3 in response to CO2. e Violin plot of mosquito speed in ROI-1 and -2 as a function of the odor treatments. f Violin plot of upwind velocity in ROI-1 and - as a function of the odor treatments. g Violin plot of tortuosity in ROI-1 and -2 as a function of the odor treatments. In all violin graphs (e–g), the CO2 treatment is colored green, the CO2 + 1-often-3-ol treatment is colored blue and the CO2 + 1-octen-3-ol + indole treatment is colored maroon. CO2 concentration: 600 ppm; 1-octen-3-ol concentration: 168 mg; indole concentration: 14.625 mg. ROI, Region Of Interest. Different lowercase letters above all violin graphs indicate significant difference at P ≤ 0.05). Median values (speed, velocity and tortuosity) are provided below each violin graph
The overlapping chemical structures between DEET and indole provided the initial impetus to test the potential blocking effect of the latter on AaegOR8. We observed a significant reduction in the current baseline of OR8 at high indole concentrations, with indole generating (R)-1-octen-3-ol-dependent hyperpolarization currents (Fig. 1c). In comparison to DEET and IR3535, these increases in current baseline were robust and exhibited different degrees of reversibility, with indole showing the highest level of insurmountability (i.e. 1-octen-3-ol failed to elicit significant responses and the switch to ND96 biffer did not restore the baseline current). This finding indicates that the affinity of indole for OR8 is higher than that of the other two tested insect repellents. We also did not observe that indole affected whole-cell currents of oocytes expressing Orco, suggesting that indole acts as an allosteric modulator of OR8.
We had previously reported on these currents, elicited by the highest concentrations of DEET, IR3535 and picaridin, without providing a molecular mechanism underlying this phenomenon [5, 7]. Recently, similar observations were reported with Cx. quinquefasciatus and An. gambiae ORs, suggesting that these hyperpolarization currents may be mediated by chloride influx [25, 26]. The reversible decrease of the baseline current in OR8-injected oocytes suggests that these hyperpolarization currents may not be generated by the activation and subsequent opening of the OR8 ion channel but, rather, may reflect a reduction of its constitutive activity. This interpretation is consistent with the observed moderate yet significant increase in baseline current.
What is the ecological role of (R)-1-octen-3-ol? OR8 is expressed in the maxillary palps of adult mosquitoes [11, 12]. It is selectively activated by nanomolar concentrations of (R)-1-octen-3-ol when expressed in Xenopus oocytes [13]. The evidence presented in this study suggests a correlative relationship between the observed repellency and one possible molecular mechanism for eliciting this behavior. We recognize that indole repellency may be generated by other molecular targets, such as the indolergic or other receptors. Indeed, indole-induced repellency may have no direct relevance to 1-octen-3-ol/OR8-mediated behavior. The high concentrations used in the present study (up to 1 M) are unlikely to be found in nature. However, at the lower used concentrations, we also observed significant repellency. Whether the same or other detection mechanisms are involved is not known. It is possible that different indole concentrations target different molecular mechanisms, as has been observed for DEET [5, 6, 27,28,29,30,31].
Indole is synthesized by bacteria [32, 33], fungi and plants [34] and released by animals [35] and may attract female mosquitoes in the contexts of animal host- [36] and oviposition-seeking [37]. In Culex mosquitoes, indole has been associated with fermented Bermuda grass infusions, which attract gravid female mosquitoes [38,39,40]. However, indole alone does not appear to act as an oviposition attractant in Ae. aegypti [19] or in An. gambiae, while it is slightly repellent to ovipositing Aedes albopictus [19].
Indole may play a role in human host-seeking behavior as well [37]. Microbiota on the skin [41] and in sweat [35] release indole and may contribute to its attractiveness. We tested the olfactory-mediated effect of multiple indole doses in the context of human host-seeking behavior and provide observational evidence that indole repels female mosquitoes (Fig. 4). Whether these doses are ecologically relevant is not known, but this observation could provide one explanation as to why different individuals exhibit different levels of attractiveness towards mosquitoes.
To test whether indole-mediated OR8 inhibition would elicit repellency, we tested its effect against blood-seeking female Ae. aegypti. Our results suggest that indole acts as an olfactory repellent in a broad range of doses (1 M–10–5 M) and loses its repellent activity at low doses (10–6 M). We used DEET as a positive control since it is a recognized and effective insect repellent. DEET probably operates through different modes of action, including interacting with both smell and taste receptors [5, 6, 27,28,29,30,31] and by reducing the volatility of odorants [9]. In our arm-in-a-cage assay, indole was not applied to the skin but rather onto a physically separate chemical holder, suggesting that the inhibitory effect of indole is not that of chemically decreasing odorant volatility (‘masking’), as has been suggested in An. gambiae [8,9,10]. The number of mosquito visits was the most significant measure of repellency. By contrast, the durations of the visits were highly variable, with DEET standing out as compared to the vehicle. However, all of the tested treatments did not show any significant differences. Taken together, these results suggest that mosquitoes were repelled before making contact with the screen, which is located immediately below the chemical source. This would be consistent with an olfactory-mediated effect, whereas once landed on the screen, olfactory information may be downplayed by the brain while other senses, such as taste or close-range chemosensation, take precedence.
The highest indole concentrations used in our behavioral experiments are unpleasant (moth ball) to the human nose and may elicit rejection from consumers. However, we have shown that indole concentrations as low as 10–5 M elicit significant repellency at short range. These concentrations are consistent with those available in the fragrance industry, which uses indoles in dilutions of ≤ 0.1% to create a floral effect in perfumes. Overall, indole demonstrates minimal toxicity at low concentrations [42]. When ingested, indole exhibits an LD50 of 1000 mg [43]. The quantity we used in our flight tunnel was 14.625 mg, while the minimal dose to repel mosquitoes in our cage assay was 146 µg (10−5 M). These quantities are well below the toxicity levels, hence the use of indole in perfumery. In addition, indole may be mixed with other volatiles to enhance this floral effect. While it is not known that perfumes containing indoles repel mosquitoes, other personal care products with plant odorants as part of their formulations have been shown to repel mosquitoes [44, 45]. Future studies on indolic perfumes might also reveal an ability to create a repelling effect.
The results of our study provide strong support that indole, a mosquito kairomone of unclear ecological significance, inhibits human host-seeking Ae. aegypti females. We also provided pharmacological evidence that the OR8/1-octen-3-ol detection pathway is a potential molecular mode of action for this inhibition. Since indole activates multiple mosquito ORs, including OR2 and OR10, the indole-mediated reduction in anemotactic flight may be caused by distinct olfactory pathways. Our observations nevertheless raise the need for additional studies on the efficacy of indole as a potential mosquito spatial repellent.
Behavior assays and electrophysiology results underlying this article will be shared on reasonable request to the corresponding author.
Schreck CE, Gilbert IH, Weidhaas DE, Posey KH.Spatial action of mosquito repellents.J Econ Entomol.1970;63:1576–8.
Article CAS PubMed Google Scholar
Khan AA, Maibach HI. A study of insect repellents. 1. Effect on the flight and approach by Aedes aegypti. J Econ Entomol. 1972;65:1318–21.
Article CAS PubMed Google Scholar
Mayer MS, James JD. Attraction of Aedes aegypti (L.): responses to human arms, carbon dioxide, and air currents in a new type of olfactometer. Bull Entomol Res. 1969;58:629–42.
Hao H, Wei J, Dai J, Du J. Host-seeking and blood-feeding behavior of Aedes albopictus (Diptera: Culicidae) exposed to vapors of geraniol, citral, citronellal, eugenol, or anisaldehyde. J Med Entomol. 2008;45:533–9.
Bohbot JD, Dickens JC. Insect repellents: modulators of mosquito odorant receptor activity. PLoS ONE. 2010;5:e12138. https://doi.org/10.1371/journal.pone.0012138.
Article CAS PubMed PubMed Central Google Scholar
Bohbot JD, Fu L, Le TC, Chauhan KR, Cantrell CL, Dickens JC. Multiple activities of insect repellents on odorant receptors in mosquitoes. Med Vet Entomol. 2011;25:436–44.
Article CAS PubMed Google Scholar
Bohbot JD, Dickens JC. Odorant receptor modulation: ternary paradigm for mode of action of insect repellents. Neuropharmacology. 2012;62:2086–95.
Article CAS PubMed Google Scholar
Syed Z, Leal W. Mosquitoes smell and avoid the insect repellent DEET. Proc Natl Acad Sci USA 2008;36:13598–603. http://www.pnas.org/cgi/content/abstract/105/36/13598.
Afify A, Betz JF, Riabinina O, Lahondère C, Potter CJ. Commonly used insect repellents hide human odors from Anopheles mosquitoes. Curr Biol. 2019;29(21):3669-3680.e5. https://doi.org/10.1016/j.cub.2019.09.007.
Afify A, Potter CJ. Insect repellents mediate species-specific olfactory behaviours in mosquitoes. Malar J. 2020;19:127.
Article CAS PubMed PubMed Central Google Scholar
[ PubMed ] Bohbot J, Pitts RJ, Kwon HW, Rutzler M, Robertson HM, Zwiebel LJ.Molecular characterization of the Aedes aegypti odorant receptor gene family.Insect Mol Biol.2007;16:525–37.
CAS PubMed PubMed Central Google Scholar
Lu T, Qiu YT, Wang G, Kwon JY, Rutzler M, Kwon H-W, et al. Odor coding in the maxillary palp of the malaria vector mosquito Anopheles gambiae. Curr Biol. 2007;17:1533–44.
Article CAS PubMed PubMed Central Google Scholar
Bohbot JD, Dickens JC. Characterization of an enantioselective odorant receptor in the yellow fever mosquito Aedes aegypti. PLoS ONE. 2009;4:e7032.
Article PubMed PubMed Central Google Scholar
Dekel A, Pitts RJ, Yakir E, Bohbot JD. Evolutionarily conserved odorant receptor function questions ecological context of octenol role in mosquitoes. Sci Rep. 2016;6:37330.
Article CAS PubMed PubMed Central Google Scholar
Gibson G, Torr S. Visual and olfactory responses of haematophagous Diptera to host stimuli. Med Vet Entomol. 1999;13:2–23.
Article CAS PubMed Google Scholar
Takken W. The role of olfaction in host-seeking of mosquitoes: a review. Int J Trop Insect Sci. 1991;12:287–95.
Takken W, Knols BG. Odor-mediated behavior of Afrotropical malaria mosquitoes. Annu Rev Entomol. 1999;44:131–57.
Article CAS PubMed Google Scholar
Lindh JM, Borg-Karlson A-K, Faye I. Transstadial and horizontal transfer of bacteria within a colony of Anopheles gambiae (Diptera: Culicidae) and oviposition response to bacteria-containing water. Acta Trop. 2008;107:242–50.
Article CAS PubMed Google Scholar
Allan SA, Kline DL. Evaluation of organic infusions and synthetic compounds mediating oviposition in Aedes albopictus and Aedes aegypti (Diptera: Culicidae). J Chem Ecol. 1995;21:1847–60.
Article CAS PubMed Google Scholar
Du Y, Millar J. Electroantennogram and oviposition bioassay responses of Culex quinquefasciatus and Culex tarsalis (Diptera: Culicidae) to chemicals in odors from Bermuda grass infusions. J Med Entomol. 1999;36:158–66.
Article CAS PubMed Google Scholar
Collins L, Blackwell A. Olfactory cues for oviposition behavior in Toxorhynchites moctezuma and Toxorhynchites amboinensis (Diptera: Culicidae). J Med Entomol. 2002;39:121–6.
Article CAS PubMed Google Scholar
Millar JG, Chaney JD, Beehler JW, Mulla MS. Interaction of the Culex quinquefasciatus egg raft pheromone with a natural chemical associated with oviposition sites. J Am Mosq Control Assoc. 1994;10:374–9.
Takken W, Loon JJA, Adam W. Effects of gonotrophic development of Anopheles gambiae (Diptera: Culicidae) on physiological and behavioural responses to human odour. J Insect Physiol. 2001;47:303–10.
Article CAS PubMed Google Scholar
R Core Team. R: a language and environment for statistical computing. Vienna: R Foundation for Statistical Computing; 2021.
Xu P, Zeng F, Bedoukian RH, Leal WS. DEET and other repellents are inhibitors of mosquito odorant receptors for oviposition attractants. Insect Biochem Mol Biol. 2019;113:103224.
Article CAS PubMed PubMed Central Google Scholar
Xu P, Choo Y-M, Chen Z, Zeng F, Tan K, Chen T-Y, et al. Odorant inhibition in mosquito olfaction. iScience. 2019;19:25–38.
Article CAS PubMed PubMed Central Google Scholar
Ditzen M, Pellegrino M, Vosshall LB. Insect odorant receptors are molecular targets of the insect repellent DEET. Science. 2008;319:1838–42.
Article CAS PubMed Google Scholar
Pellegrino M, Steinbach N, Stensmyr MC, Hansson BS, Vosshall LB. A natural polymorphism alters odour and DEET sensitivity in an insect odorant receptor. Nature. 2011;478:511–4.
Article CAS PubMed PubMed Central Google Scholar
Sanford JL, Shields VDC, Dickens JC. Gustatory receptor neuron responds to DEET and other insect repellents in the yellow-fever mosquito Aedes aegypti. Naturwissenschaften. 2013;100:269–73.
Article CAS PubMed Google Scholar
Dennis EJ, Goldman OV, Vosshall LB. Aedes aegypti mosquitoes use their legs to sense DEET on contact. Curr Biol. 2019;29:1551-1556.e5.
Article CAS PubMed PubMed Central Google Scholar
Grant GG, Estrera RR, Pathak N, Hall CD, Tsikolia M, Linthicum KJ, et al. Interactions of DEET and novel repellents with mosquito odorant receptors. J Med Entomol. 2020;57:1032–40.
Article CAS PubMed Google Scholar
Elgaali H, Hamilton-Kemp TR, Newman MC, Collins RW, Yu K, Archbold DD. Comparison of long-chain alcohols and other volatile compounds emitted from food-borne and related Gram positive and Gram negative bacteria. J Basic Microbiol. 2002;42:373–80.
Article CAS PubMed Google Scholar
Chen G, Zhang R-R, Liu Y, Sun W-B. Spore dispersal of fetid by feces of mycophagous insects. J Chem Ecol. 2014;40:893–9.
Article CAS PubMed Google Scholar
Turlings TC, Tumlinson JH, Heath RR, Proveaux AT, Doolittle RE. Isolation and identification of allelochemicals that attract the larval parasitoid, Cotesia marginiventris (Cresson), to the microhabitat of one of its hosts. J Chem Ecol. 1991;17:2235–51.
Article CAS PubMed Google Scholar
Meijerink J, Braks MAH, Brack AA, Adam W, Dekker T, Posthumus MA, et al. Identification of olfactory stimulants for Anopheles gambiae from human sweat samples. J Chem Ecol. 2000;26:1367–82.
Blackwell A, Johnson S. Electrophysiological investigation of larval water and potential oviposition chemo-attractants for Anopheles gambiae s.s. Ann Trop Med Parasitol. 2000;94:389–98.
Article CAS PubMed Google Scholar
Cork A. Olfactory basis of host location by mosquitoes and other Haematophagous Diptera. In: Bock GR, Cardew G, editors. Olfaction in mosquito-host interactions Ciba foundation symposium 200. Chichester: Wiley and Sons; 1996. p. 71–88.
Millar JG, Chaney JD, Mulla MS. Identification of oviposition attractants for Culex quinquefasciatus from fermented Bermuda grass infusions. J Am Mosq Control Assoc. 1992;8:11–7.
Blackwell A, Mordue A, Hansson B. A behavioural and electrophysiological study of oviposition cues for Culex quinquefasciatus. Physiol Entomol. 1993;18:343–8.
Beehler J, Millar J, Mulla M. Synergism between chemical attractants and visual cues influencing oviposition of the mosquito, Culex quinquefasciatus (Diptera: Culicidae). J Chem Ecol. 1993;19:635–44.
Article CAS PubMed Google Scholar
Bernier UR, Kline DL, Barnard DR, Schreck CE, Yost RA. Analysis of human skin emanations by gas chromatography/mass spectrometry. 2. Identification of volatile compounds that are candidate attractants for the yellow fever mosquito (Aedes aegypti). Anal Chem. 2000;72:747–56.
Article CAS PubMed Google Scholar
US Environmental Protection Agency (USEP). Indole PC Code 025000. Washington DC: US Environmental Protection Agency; 2009. https://nepis.epa.gov/Exe/ZyPDF.cgi/P1004V9K.PDF?Dockey=P1004V9K.PDF. Accessed 3 Nov 2022.
Smyth HF Jr, Carpenter CP, Well CS, Pozzani UC, Striegel JA. Range-finding toxicity data: list VI. Am Ind Hyg Assoc J. 1962;23:95–107.
Article CAS PubMed Google Scholar
Verhulst NO, Weldegergis BT, Menger D, Takken W. Attractiveness of volatiles from different body parts to the malaria mosquito Anopheles coluzzii is affected by deodorant compounds. Sci Rep. 2016;6:27141.
Article CAS PubMed PubMed Central Google Scholar
Zeng F, Xu P, Tan K, Zarbin PHG, Leal WS. Methyl dihydrojasmonate and lilial are the constituents with an “off-label” insect repellence in perfumes. PLoS ONE. 2018;13:e0199386.
Article PubMed PubMed Central Google Scholar
The authors wish to thank Drs Michael J. Gutnick (The Hebrew University of Jerusalem), Andreas Neef (The University of Göttingen) and Gregory Pask (Bucknell University) for their recommendations and insights on the pharmacological study of ligand-gated ion channels. This research was supported by the ISRAEL SCIENCE FOUNDATION (Grant No. 719/21).
This work was supported by the Israel Science Foundation [719/21].
Amir Dekel and Evyatar Sar-Shalom contributed equally to this work
Department of Entomology, The Robert H. Smith Faculty of Agriculture, Food and Environment, The Hebrew University of Jerusalem, 76100, Rehovot, Israel
Amir Dekel, Evyatar Sar-Shalom, Yuri Vainer, Esther Yakir & Jonathan D. Bohbot
You can also search for this author in PubMed Google Scholar
You can also search for this author in PubMed Google Scholar
You can also search for this author in PubMed Google Scholar
You can also search for this author in PubMed Google Scholar
You can also search for this author in PubMed Google Scholar
The concept of this study was conceived by EY. JDB and ESS designed the study. AD carried out the pharmacological characterization. YV contributed to the pharmacological characterization. ESS conducted all the behavioral experiments. JDB, AD and ESS analyzed the data. JDB and ESS wrote the manuscript. All authors read and approved the final manuscript.
Correspondence to Jonathan D. Bohbot.
The Authors consent to publication of the work in Parasites and Vectors.
The authors declare that they have no competing interests.
Springer Nature remains neutral with regard to jurisdictional claims in published maps and institutional affiliations.
: Figure S1. Diagrams of the odor delivery system. A The odor delivery system is composed of interlocking top and bottom rings attached to a removable chemical holder (overview, top, bottom and side views are provided). Dimensions are provided in millimeters. B The rings are locked in place across the glove of the person conducting the experiment. The glove within the inner area of the ring is removed, exposing the skin surface. A plastic net is intercalated between the rings and serves to physically protect the skin from mosquito bites.
: Hand rings.stl. File format for 3D-printing of the two complementary hand rings.
: Chemical holder.stl. File format for 3D-printing of the chemical holder component.
: Table S1. Scripts and functions used to calculate flight parameters.
: Table S2. EC50 values (Log [M]) of AaegOR8-Orco concentration-response relationships.
Additional file 6: Video S1. Representative videos of arm-in-cage assays. Side-by-side recorded sessions of the first 5 min of a control experiment (vehicle) and an indole (0.1 M) treatment
: Figure S2. High indole concentrations exhibit significant repellency over time. Cumulative number of mosquito landings per elapsed minute (see Fig. 4b). Points represented are mean ± SEM (n = 3). Statistical significance was determined using a non-parametric test followed by a Dunn’s multiple comparisons test (P values shown on the histograms).
: Figure S3. Representative single flight trajectories of female mosquitoes exposed to CO2, 1-octen-3-ol and indole, respectively. A Schematic of the flight tunnel and the overall region of interest (ROI) located between 500 and 1300 mm on the X-axis (shaded gray box). The three odor treatments are color-coded. B Example trajectories of mosquitoes exposed to CO2 or to a combination of CO2 + 1-octen-3-ol, or CO2 + 1-octen-3-ol + indole. These trajectories were recorded in the ROI within the flight tunnel and projected into each of the three 2-dimensional planes (from left to right, Y-X, Z-X, and Z-Y). Speed is color-coded according to the speed index. The last coordinate of the trajectory is shown in black and marked with an arrow. The dashed line indicates trajectory segments outside the ROI.
Open Access This article is licensed under a Creative Commons Attribution 4.0 International License, which permits use, sharing, adaptation, distribution and reproduction in any medium or format, as long as you give appropriate credit to the original author(s) and the source, provide a link to the Creative Commons licence, and indicate if changes were made. The images or other third party material in this article are included in the article's Creative Commons licence, unless indicated otherwise in a credit line to the material. If material is not included in the article's Creative Commons licence and your intended use is not permitted by statutory regulation or exceeds the permitted use, you will need to obtain permission directly from the copyright holder. To view a copy of this licence, visit http://creativecommons.org/licenses/by/4.0/. The Creative Commons Public Domain Dedication waiver (http://creativecommons.org/publicdomain/zero/1.0/) applies to the data made available in this article, unless otherwise stated in a credit line to the data.
Dekel, A., Sar-Shalom, E., Vainer, Y. et al. The oviposition cue indole inhibits animal host attraction in Aedes aegypti (Diptera: Culicidae) mosquitoes. Parasites Vectors 15, 422 (2022). https://doi.org/10.1186/s13071-022-05545-8
DOI: https://doi.org/10.1186/s13071-022-05545-8
Anyone you share the following link with will be able to read this content:
Sorry, a shareable link is not currently available for this article.
Provided by the Springer Nature SharedIt content-sharing initiative
By using this website, you agree to our Terms and Conditions, California Privacy Statement, Privacy statement and Cookies policy. Manage cookies/Do not sell my data we use in the preference centre.
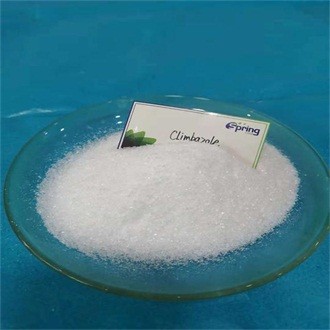
Adbac 50% © 2022 BioMed Central Ltd unless otherwise stated. Part of Springer Nature.